Introduction
Zinc is a crucial micronutrient required for normal growth and development in all living beings. Zinc acts as a cofactor for nearly 300 (10–15%) cellular enzymes, which include superoxide dismutase (SOD) and tryptophan-synthetase (Suganya et al., 2020). SOD is responsible for converting superoxide to H2O2, while tryptophan-synthetase catalyzes the transformation of serine and indol-3-glycerol phosphate into tryptophan, both of which occur during tryptophan biosynthesis (Schlee et al., 2013).
The required amount of zinc for crops varies from 5 to 100 mg/kg, depending on the crop. Examples of crops that require zinc include okra, wheat, tomato, and maize (Baruah et al., 2019; Eshaghi et al., 2019). Zinc is essential for various cellular processes, such as indole metabolism, membrane functioning, carbonic anhydrase, RNA polymerases, macromolecule metabolism, alcohol dehydrogenase, chloroplast production, and others (Nieder et al., 2018; Salehi et al., 2019).
Plants absorb zinc in the dissolved form as Zn2+, although a significant portion of zinc in the soil is insoluble. Several physical and chemical soil variables induce zinc shortage in staple foods, including elevated bicarbonate and phosphate levels, high alkalinity, low total zinc content, and high calcite content (Schlee et al., 2013; Nieder et al., 2018; Eshaghi et al., 2019; Salehi et al., 2019). The low zinc content in India's agricultural soil adversely affects the country's agricultural output (Baruah et al., 2019).
Plant growth and health can be improved by adding microbial inoculants, vermicompost, organic compost, and chemical fertilizers, which also enhance soil composition and consistency. However, the widespread use of chemical fertilizers in conventional farming practices can lead to a loss of soil qualities and a detrimental effect on soil microflora. To reduce zinc demand in plant life and agricultural production, exceptional bacteria that can potentially solubilize inaccessible forms of insoluble zinc into soluble forms can be isolated as a potential technique.
Tomatoes are now being recognized for their potential in preventing certain human ailments due to their high content of carotenoids and lycopene. Lycopene, an unsaturated alkylic molecule, is thought to be an active component in protecting against cancer, cardiovascular disease, and delaying cellular aging (Salehi et al., 2019). Tomatoes also contain various nutrients and secondary metabolites beneficial to human health, such as lycopene, organic acids, B-carotene, flavonoids, phenolics, vitamins C and E, minerals, and chlorophyll (Li et al., 2021).
To enhance soil health and consistency, synthetic fertilizers, natural manure, vermicast, or microbiological inoculants can be added to stimulate plant growth and improve soil health (Nieder et al., 2018; Karagoz and Dursun, 2020). Using cow dung as a fertilizer is an environmentally friendly alternative to synthetic fertilizers that helps maintain soil attributes by providing necessary nutrients over a more extended period and preserving the microflora of soil (Suganya et al., 2020; Ahmed et al., 2021). The microorganisms available in cow dung may also work as plant growth-promoting (PGP) agents by providing phytohormones and mineralizing unutilized minerals. Therefore, selecting relevant zincsolubilizing bacteria (ZSB) from cow dung is the foremost and most crucial step in the current work.
This step involves detecting cow dung bacteria exhibiting PGP properties, zinc solubilizing efficiency, and examining tomato growth parameters. The present work aims to isolate and screen ZSB from cow dung and assess their potential PGP attributes, such as phytohormone production (indole acetic acid (IAA)), iron-chelator production (siderophore), aminocyclopropane-1-carboxylate (ACC) deaminase activity, and inorganic phosphate solubilization in the laboratory. Furthermore, the isolated Zn-solubilizing PGP bacteria were tested for their potential to improve plant growth in vivo on tomato seeds in a pot assay, and plant growth parameters, such as plant development, stem girth, fruit numbers, and fruit Zn content, were recorded.
Methods
Screening of Zn solubilizing bacteria from cow dung
To isolate and screen the ZSB, cow dung was collected from the Bhojia Institute of Life Sciences (BILS) in Baddi, Himachal Pradesh, India (31°2′37.9716″N, 76°43′3.1296″E). One gram of cow dung sample was thoroughly pulverized and utilized in the study. A 100 μl diluted sample (10−5 to 10−6 dilution) was disseminated on optimized King's B media (protease peptone 20 g/l, K2HPO4 1.5 g/l, MgSO4 · 7H2O 1.5 g/l, glycerol 10 ml/l, and agar 15 g/l) supplemented with an insoluble zinc source (0.1% ZnO or 0.1% ZnCO3) individual medium (Karnwal, 2021b). The plates were then incubated at 28±2°C for 2 days. Bacterial isolates that exhibited Zn solubilization and formed halo zones around the bacterial colonies were selected to assess zinc solubilization and plant growth promotion activities. Eleven positive strains for Zn solubilization were obtained in pure form and stored as 30% glycerol stocks at −20°C.
Zinc-solubilization analysis
To determine the Zn solubilization, Zn solubility index (SI), and Zn solubility efficiency (SE) of selected ZSB, a higher concentration of insoluble Zn source (1% ZnO or 0.1% ZnCO3) was used. After 24 h of incubation at 28±2° C, the bacterial isolates were spotted on Zn source (ZnO and ZnCO3) enriched King's B plates and cultivated for five days at 28±2°C. Bacterial isolates with the maximum solubility of insoluble Zn were separated, and the diameters of the solubility zones were measured. The SI and SE of bacterial isolates were calculated (Goteti et al., 2013) using the following formulas:
The residual Zn content in the bacterial suspension was measured using atomic absorption spectroscopy (AAS) at 5, 10, and 15 days intervals to estimate the Zn content. The AAS findings presented zinc amounts in parts per million. The pH of the culture filtrates and control (uninoculated) samples were also assessed at 5, 10, and 15 days after inoculation under acidic and alkaline conditions. The pH of the nutrient broth with Zn source was adjusted using 1 M HCl and 1 M NaOH during the experiment to maintain a pH of 7. Zinc-solubilizing bacterial isolates were then inoculated on the zinc-supplemented medium and incubated at 28±2°C for 24 h for solubilization.
Assessment of isolates for their PGP activities
Eleven bacterial isolates were evaluated for their ability to promote plant growth, including their phytohormone production (i.e., IAA), iron-chelator production (siderophore), ACC deaminase activity, and inorganic phosphate solubilization. The Salkowski reagent was used to qualitatively and quantitatively analyze IAA production by the 11 bacterial isolates (Palaniappan et al., 2010 ; Shao et al., 2015).
The Chrome-azurol S agar media was used to determine siderophore production and quantification, as described by Schwyn and Neilands (1987). The bacterial ability to produce ACC deaminase was determined using the sole carbon source, ACC, in a supplemented media, following the approach of Dworkin and Foster (1958).
Pathogenicity of isolates
Two isolates were selected based on their Zn solubilization and PGP activities for pathogenicity testing. The selected isolates' pathogenicity was determined using blood-agar plates to assess their hemolytic activity. The blood-agar plates were prepared using sterilized nutrient agar (Hi-media) medium. For the blood agar, sterilized nutrient agar was cooled to 45–50°C and aseptically mixed with 6% sterile defibrinated sheep blood purchased from a nearby slaughterhouse. The mixture was then plated in sterile Petri plates under laminar airflow. These plates were spotted with the bacterial cultures and incubated at 28±2°C for 48 h to determine the ability of the bacterial isolates to hemolyze red blood cells. The plates were examined for α (greenish), β (transparent), and γ hemolysis (no zone) as described by Jayaseelan et al. (2014).
Characterization and phylogenetic analysis of isolates
The selected bacterial isolates were characterized microscopically and biochemically using Bergey's manual of determinative bacteriology (Holt et al., 1994). To isolate bacterial DNA from a pure bacterial culture, the modified CTAB approach was used (Karnwal, 2021a). Universal PCR primers were used for 16S rRNA gene amplification of bacterial isolates, as described by Karnwal (2021a). The sequenced 16S rRNA gene was aligned with the GENBANK database using the BLAST tool to match with the available type strain sequence and submitted to the GENBANK database with OL960375 and OL960472 accession numbers.
Bacterial consortium analysis
Both ZSB were evaluated for their compatibility as a microbial consortium. To develop a bacterial consortium, Luria Bertani plates were spread with 1000 μl of one isolate and incubated for 15 min at 28±2°C. This was followed by inoculation with another bacterial culture and cultivation at 28±2°C for 24–48 h, as described by Baliyan et al. (2018) and Wang et al. (2021).
Pot assay
The study investigated the effects of two selected isolates (CDS7 and CDS21) on the growth and development of tomato plants. To bacterize healthy tomato seeds, the approach described by Weller and Cook (1983) was followed. Tomato seeds (Solanum lycopersicum L.) were obtained from IARI, Pusa, New Delhi, India, and surface sterilized using 95% ethyl alcohol for 1 min and 7% sodium hypochlorite for 3 min. The seeds were then washed with sterilized distilled water (DW) three times to remove the remaining sterilizing chemicals. Surface sterilized seeds were dried aseptically under a laminar airflow hood. The bacterial culture in nutrient agar medium was freshly grown (24-h incubation) and placed in a cooling centrifuge at 10 000 g for 10 min to isolate the bacterial pellet.
Subsequently, the bacterial pellets were suspended in sterile DW and blended with 1% carboxy-methyl-cellulose to form the final solution, referred to as bacterial slurry. The surface of the sterilized seeds was coated with the bacterial slurry. For the pot study, a randomized block pattern with eight seeds per pot was used, with the seeds inoculated separately with both bacterial isolates and a consortium of both bacterial slurries, and no bacterial slurry was used as the control. Sterilized soil was used in triplicates for each treatment, as follows:
T1 treatment: control;
T2 treatment: (seeds + CDS7);
T3 treatment: (seeds + CDS21);
T4 treatment: (seeds + consortium).
After 60 days of planting (DAP), root and shoot (fresh/dry) weight, root length, and plant height were measured. The tomato fruits were harvested after 60 days of the plantation, and their Zn concentrations were determined in each treatment.
Plant growth measurements
At maturity, which was 60 days after planting, the plants were collected, and data on plant development, stem girth, fruit numbers, and fruit Zn content were recorded. Physiological data were measured during the growth period. The fresh shoot and root biomass were determined using an electronic balance after harvesting. The shoots and roots were then dried in the oven at 72°C until they reached a consistent weight, and their dry weights were measured.
Zn content analysis
To assess the Zn content in the tomato fruit samples from each treatment, the samples were first dried at 70°C for 48 h and then pulverized. One gram of the pulverized fruit sample was digested in 10 ml of HNO3: HClO4 (2 :1 v/v) for Zn assessment, as described by Dhaked et al. (2017). The filtered solution of Whatman's filter paper-42 was subjected to spectrophotometric analysis using AAS to estimate the total Zn amount, following the method described by Karnwal (2021b). The obtained data were statistically analyzed. The Zn concentration of the soil used for the experiment was also tested before planting and after 60 days of planting.
Results
In this study, Pseudomonas spp. were identified from cow dung. Out of 35 bacterial isolates tested based on their ability to solubilize zinc sources (ZnO and ZnCO3), only 11 isolates were effective and were labeled as CDS3, CDS7, CDS9, CDS11, CDS14, CDS17, CDS21, CDS25, CDS26, CDS27, and CDS32. These 11 isolates were examined for morphological and biochemical characteristics, which are presented in Table 1.
Table 1
Microscopic and biochemical characterization of isolated bacterial strains
Molecular identification of the CDS7 and CDS21 isolates
The results of 16S rRNA gene sequencing of CDS7 and CDS21 showed that they had 97.17 and 95.33% similarity, respectively, with Pseudomonas kilonensis 520-20 and Pseudomonas chlororaphis DSM 50083. The 16S rDNA sequences for both strains, P. kilonensis CDS7 and P. chlororaphis CDS21, were deposited in the GenBank (NCBI) repository with the accession IDs OL960375 and OL960472, respectively. A phylogenetic tree was created for both strains, which is shown in Figure 1.
In-vitro zinc solubilization assessment
The ability of two bacterial isolates to solubilize zinc was evaluated by measuring the transparent zone around their bacterial colony growth. All 11 isolates tested in this study showed different levels of solubility of zinc in media enriched with ZnO and ZnCO3. CDS27 exhibited the largest zone of Zn solubilization (32 mm) on a medium supplemented with zinc oxide, followed by CDS7 (26 mm) and CDS21 (25 mm) after seven days of incubation. CDS14 and CDS26 also displayed significant Zn solubilization with a 20 mm zone of solubilization in media supplemented with an insoluble ZnO (Fig. 2A). However, in media enriched with zinc carbonate, comparable solubilization by CDS27 (24 mm), CDS21 (22 mm), and CDS7 (18 mm) was observed. After 15 days of incubation, the results of AAS showed that CDS7 (32.1 mg/l) had the maximum solubilization of zinc oxide, followed by CDS21 (23.7 mg/l) and CDS27 (21.8 mg/l). In contrast, in media supplemented with zinc carbonate, CDS7 (19.4 mg/l) showed the highest zinc solubilization, followed by CDS21 (17.2 mg/l) and CDS27 (15.2 mg/l), as shown in Figure 3A. Compared to the other six isolates, CDS7 and CDS21 demonstrated higher Zn solubilization efficiency (ZnO) with 650 and 416.67%, respectively, followed by CDS27 (390.24%) and CDS11 (320.00%). Although there was variability in solubilization efficiency in media supplemented with ZnCO3, CDS7, and CDS21 exhibited better results, followed by CDS27 and CDS17, as depicted in Figure 2C.
Fig. 2
Zn solubilization assessment of isolated bacterial isolates after 7 days of incubation (DAI): A) solubilization zone, B) solubilization index, and C) solubilization efficiency
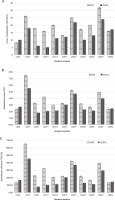
Fig. 3
Zn solubilization efficiency of isolated bacterial strains on ZnO and ZnCO3 supplemented media A) AAS results for Zn solubilization by isolated bacterial strains after 15 DAI; B) ZnO-supplemented media pH after 7 DAI depending on Zn solubilization efficiency; C) ZnCO3-supplemented media pH after 7 DAI depending on Zn solubilization efficiency
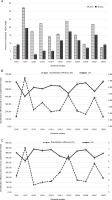
The results of the alkaline and acidic Zn solubilization assessments indicated that the pH of media supplemented with ZnO and ZnCO3 was reduced to 4.6 and 5.4, respectively, after 15 days of incubation, compared to the initial pH of 7. In the presence of zinc oxide, the cellfree culture of CDS7 was found to have a pH of 4.6, followed by CDS21 with a pH level of 5.1 (Fig. 3B). In contrast, in the culture-free supernatant enriched with ZnCO3-modified media, corresponding isolates had reported pH values of 5.1 and 5.4, respectively (Fig. 3C). The lower pH indicates a higher level of acidity.
Assessment of PGP characteristics
Microorganisms that have a positive impact on plant growth play a crucial role in plant development through direct or indirect mechanisms, including the production of phytohormones such as indole butyric acid, indole acetic acid, gibberellic acid, cytokines, organic acids, siderophores, ACC deaminase, hydrogen cyanide (HCN), and mineral mineralization of phosphorus and zinc. In this study, it was determined in vitro that all 11 Zn solubilizing isolates had PGP characteristics, such as phosphate solubilization, IAA generation, siderophore synthesis, and ACC deaminase, as shown in Table 2. CDS7 had the highest phosphate solubilization ability (287.2 μg/ml), followed by CDS21 (217.7 μg/ml) and CDS27 (194.47 μg/ml) among the 11 isolates (Table 2). Isolates CDS3, CDS9, CDS25, CDS26, and CDS32 did not exhibit any phosphate solubilization efficiency. Additionally, the IAA production experiment indicated the potential of IAA production by six Zn solubilizing strains (CDS3, CDS7, CDS9, CDS21, CDS27, and CDS32), with IAA production ranging between 9.8 and 22.1 μg/ml after 24–72 h of incubation. CDS7 and CDS3 produced the highest levels of IAA, 22.1 and 15.71 μg/ml, respectively (Table 2). CDS21, CDS27, and CDS9 produced 14.8, 13.5, and 13.7 μg/ml of IAA, respectively. Out of the 11 isolates tested for siderophore production, four isolates (CDS7, CDS21, CDS26, and CDS32) were found to be positive (Table 2). The maximum levels of ACC deaminase activity were observed after 8 days of culturing for CDS9, followed by CDS25 and CDS27.
Table 2
Comparative analysis of bacterial isolates based on PGP characteristics, n = 3
Isolates | Production of siderophore | ACC deaminase | Indole acetic acid production | IAA quantity [μg/ml] | Solubilization of phosphate | Phosphate solubilization [μg/ml] |
---|---|---|---|---|---|---|
CDS3 | – | + | +++* | 15.7±0.12 | – | 0±0 a |
CDS7 | +++ | + | +++ | 22.1±0.43 | +++ | 287.2±6.8 |
CDS9 | – | +++ | + | 13.7±0.44 | – | 0±0 |
CDS11 | – | + | – | 0±0 | + | 65±3.6 |
CDS14 | – | + | – | 0±0 | + | 163±4.8 |
CDS17 | – | – | – | 0±0 | + | 76±2.1 |
CDS21 | +++ | – | + | 14.8±1.6 | +++ | 217.7±8.8 |
CDS25 | – | +++ | – | 0±0 | – | 0±0 |
CDS26 | +++ | – | – | 0±0 | – | 0±0 |
CDS27 | – | +++ | + | 13.5±0.55 | + | 197.47±3.8 |
CDS32 | +++ | – | + | 9.8±2.4 | – | 0±0 |
Pot experiment and analysis of tomato biological growth parameters
Results from the pot study demonstrated that seedlings treated with CDS7 (T2) produced significantly more fruits per plant (41.49/plant) and had significantly greater plant height (63.16 cm), stem girth (22.00 cm), fresh and dry shoot weight (38.03 and 8.06 g, respectively), and root weight (17.97 and 4.68 g, respectively) than seedlings treated with CDS21 (T3) after 60 days of cultivation. Moreover, the T2 treatment yielded the highest number of fruits (41.49), followed by the T4 treatment with 37.79 fruits per plant after 60 DAP. Zinc levels in the fruits showed a significant increase in the inoculated plants compared to the control (T1), as shown in Table 3. The maximum Zn content was found in the T2 and T4 treatments, with 3.13 and 2.36 mg/100 g of fruit, respectively, followed by the T3 treatment with 2.24 mg/100 g. This finding suggests a 45–60% increase in fruit yield and a 255–398% increase in fruit Zn content, which could be attributed to the inoculation of bacterial strains capable of producing phytohormones, organic acids, siderophores, and performing P-solubilization.
Table 3
Results of tomato growth attributes after 60 days of planting with different treatments, n = 3
Discussion
Zinc deficiency is a prevalent micronutrient deficiency in crops, resulting in significant agricultural production losses (Upadhayay et al., 2022). Zinc is a crucial micronutrient for plant growth and Upadhyay plays a significant role in their development. Biofortification of crops has gained considerable attention in enhancing mineral biofortification in plants and their products. Biofortification presents a realistic and cost-effective option for low-income nations to produce food with higher Zn content, as biofortified foods are readily accessible and affordable compared to industrially fortified food (Sharma et al., 2015; Prasad et al., 2019).
This study aimed to investigate the biofortification of zinc in plants by promoting their growth. The availability of dissolved zinc in the soil is limited, which reduces the soil’s nutritional value (Palaniappan et al., 2010; Karnwal, 2021b). Using zinc mobilizing bacteria as bioinoculants can aid in providing adequate zinc content and rendering it from an inaccessible to an accessible form, which is essential for plant development. This study included the isolation, characterization, and screening of bacteria from cow dung for their Zn mobilization and PGP activity. Two insoluble Zn sources (ZnO and ZnCO3) were used to assess the Zn solubilizing potential of the isolates. ZnO and ZnCO3 are the most cost-effective forms of zinc since they supply 50–80% zinc, more than zinc sulfate (ZnSO4), which only delivers 33% zinc (Hafeez et al., 2013). The primary challenge with using ZnO and ZnCO3 in fields is their low solubility and bioavailability compared to ZnSO4, which is considerably more soluble.
In this study, a total of 35 bacterial isolates were examined for their ability to solubilize zinc from cow dung samples obtained from dairies near BILS. Among the isolates tested, CDS21 and CDS7 were found to be the most efficient ZSB strains. These strains were further investigated for their ability to promote plant growth through the synthesis of IAA, siderophores, P-solubilization, and ACC deaminase activity.
This study evaluated the Zn solubilizing and PGP capabilities of cow dung microflora and found them to be highly beneficial in reducing media pH and increasing Zn mobilization. Previous research (Kumar et al., 2019) has shown that ZSB inoculation can stimulate shoot and root development by releasing Zn-chelating residues. Microorganisms' ability to solubilize rock phosphate has also played a vital role in increasing the bioavailability of rock phosphate to plants (Ahmed et al., 2021). The Zn solubilizing activity of Pseudomonas sp. (ZSB-S-2) was previously reported to increase Zn release from ZnO (Shaikh and Saraf, 2017). In the current study, CDS7 and CDS21 exhibited higher Zn solubilization efficiency (ZnO) of 650 and 416.67%, respectively, followed by CDS27 (390.24%) and CDS11 (320.00%). These findings are consistent with earlier reports where maximum Zn solubilization was achieved in ZnO and ZnCO3-supplemented media (Mumtaz et al., 2017; Mumtaz et al., 2019; Bhatt and Maheshwari, 2020; Karnwal, 2021a). These findings are also supported by the study of Ramesh et al. (2014), where strains of Bacillus aryabhattai were found to have Zn solubilizing efficiency of up to 175%.
The results of our study are consistent with previous findings. For example, Sharma et al. (2011) reported that the zinc concentration of different Bacillus spp. ranged between 2.23 and 4.87 μg/ml. Costerousse et al. (2017) found that Streptomyces narbonensis strain 68 exhibited good zinc-solubilizing ability, with a zinc content of 272.2 μg/ml in a solubilization assay medium containing ZnO. Streptomyces nanhaiensis strain YM4 showed a zinc solubilization of 41.66 μg/ml in a minimal medium supplemented with 0.1% (w/v) ZnO (Patel and Thakker, 2020). In another study, Yadav et al. (2022) found that different rhizobacterial strains produced varying levels of zinc content in a liquid medium, ranging from 2.47 to 6.1 μg/ml.
As mentioned in a previous study (Shaikh and Saraf, 2017), pH plays a critical role in achieving maximal solubilization of zinc. Mumtaz et al. (2017) found a direct relationship between the drop in pH of the medium and the increase in zinc availability. In the study by Yadav et al. (2022), it was reported that rhizobacterial isolates with zinc solubilizing capability were able to lower the pH of the medium from 7 to 4.5. The present study also found that all 11 isolates were able to decrease the pH of the media, with pH values between 4.6 and 5.1 being optimal for maximal solubilization of ZnO by CDS7 and CDS21 (650 and 416.6% solubilization efficiency). For optimum solubilization of ZnCO3 by CDS7 and CDS21, pH 5.1 and 5.4 were found to be optimal (with solubilization efficiencies of 450 and 366.6%, respectively), preceded by pH 5.6 for CDS27 (with a solubilization efficiency of 292.6%). The findings of this study are consistent with the earlier reports by Mumtaz et al. (2017) which showed a direct relationship between the decrease in pH of the medium and the increase in zinc availability and with the findings of Yadav et al. (2022) regarding the ability of zinc-solubilizing rhizobacterial isolates to lower the pH from 7 to 4.5.
PGP bacteria play a vital role in plant growth through various mechanisms, including the production of IAA, organic acids, HCN, siderophores, ACC deaminase activity, and mineralization of various minerals such as P liquefaction and Zn solubilization, all of which are beneficial characteristics for plant development (Qessaoui et al., 2019).
In previous studies, microbial inoculants with the ability to produce IAA, Zn, and P solubilization have been shown to play a crucial role in promoting plant growth and crop yields, particularly in okra (Karnwal, 2021b). Pseudomonas strains have been identified as having the ability to solubilize phosphate, synthesize siderophores, ammonia, and indole-3-acetic acid, and colonize the roots of tomato plants (Qessaoui et al., 2019). In the current investigation, the inoculation of ZSB led to enhanced fruits per plant, plant height, stem girth, fresh and dry shoot weight, fresh and dry root weight, and Zn content in fruits after 60 days of cultivation (35.72–41.49/plant, 58.80 –63.16 cm, 19.16–22.00 cm, 32.61–38.03 g, 6.64–8.06 g, 17.42–18.94 g, 4.06–7.48 g, 2.24–3.13 mg/100 g, respectively).
The previous study by Vidyashree et al. (2018) showed that Bacillus sp. (PAN-TM1) had a direct growth-promoting impact on tomato seedlings, with the best growth attributes including plant height (94.1 cm), number of branches per plant (27.6), stem girth (2.05 cm), and total biomass (100–30.7 g). Meanwhile, Zaheer et al. (2016) found that inoculating chickpeas with Pseudomonas strain AZ5 or Bacillus strain AZ17 resulted in significant increases in growth parameters compared to noncontrols. However, Pseudomonas AZ5 strain treated plants had a higher grain production and straw mass compared to Bacillus AZ17 strain treated crops. Similar findings were reported by Vidyashree et al. (2018), where increased Zn solubility in the presence of B. aryabhattai and Bacillus sp. (PAN-TM1) enhanced tomato development through the release of several organic acids and the production of PGP molecules such as auxins, gibberellic acid, and siderophore. In another study, Fan et al. (2017) observed that Bacillus amyloliquefaciens IN937a and Bacillus pumilus T4 promoted tomato growth, significantly impacting tomato development by boosting dry shoot mass and shoot nitrogen absorption ability. PGPR inoculation improved shoot N absorption from 10.2 to 39.0% compared to control. Additionally, Vaid et al. (2014) reported rice growth promotion and 42.7% increased zinc nutrition of grains when inoculated with zinc-solubilizing bacteria. Several reports have high-lighted the role of PGP microbes in promoting plant growth parameters through multiple mechanisms (Zaheer et al., 2016; Fan et al., 2017; Vidyashree et al., 2018). To the best of our understanding, the isolates P. kilonensis CDS7 and P. chlororaphis CDS21 used in this investigation have not yet been documented for their zinc-solubilizing potential, zinc biofortification, and role in enhancing the growth of tomato plants. However, our study showed that these strains increased tomato plant root and shoot length, dry weight, and zinc content compared to the ideal control.
Conclusion
It is noteworthy that this study is the first to report on the screening of Zn solubilizing PGP bacteria isolated from cow dung. The results of the study demonstrate that the application of Zn solubilizing PGP bacteria to tomato seedlings leads to improved growth and increased production of tomatoes. Moreover, the various PGP characteristics of the isolates suggest that they have the potential to be used as microbial biofertilizers to enhance tomato production while also addressing nutrient deficiencies, which is crucial for long-term agricultural sustainability.